16 Fallibilism
Let’s recap. We’ve discussed three problems:
- the problem of sensations: there is no guarantee that our senses convey the exact picture of things as they really are;
- the problem of induction: no matter how many confirming instances are observed, inductive generalizations remain fallible;
- the problem of theory-ladenness: the results of experiments and observations are shaped by our accepted theories.
Together, these three problems suggest that synthetic propositions cannot be established beyond any reasonable doubt. Thus, we arrive at the conception of fallibilism, which holds that no synthetic proposition is infallible and, thus, empirical knowledge cannot be absolutely certain.
Fallibilism is the currently accepted conception concerning the possibility of absolute empirical knowledge. However, just because science doesn’t know everything with absolute certainty, doesn’t mean that it doesn’t know anything (there are conclusions about nature that we do know to some degree of certainty). This is a common tactic used by those who oppose science and scientific evidence. This tactic of “Science doesn’t know everything” is used to promote alternative ideas such as New Age crystal therapy and other pseudoscientific nonsense. One technique to check whether something is scientific or not is to use Popper’s (1959/2002) definition of falsifiability: falsifiability involves seeing whether a proposition could be rendered false under some testable circumstance. Sagan (1995) provides a wonderful example to illustrate this:
The Dragon in my Garage
“A fire-breathing dragon lives in my garage”
Suppose I seriously make such an assertion to you. Surely, you’d want to check it out, see for yourself. There have been innumerable stories of dragons over the centuries, but no real evidence. What an opportunity!
“Show me,” you say. I lead you to my garage. You look inside and see a ladder, empty paint cans, an old tricycle — but no dragon.
“Where’s the dragon?” you ask.
“Oh, she’s right here,” I reply, waving vaguely. “I neglected to mention that she’s an invisible dragon.”
You propose spreading flour on the floor of the garage to capture the dragon’s footprints.
“Good idea,” I say, “but this dragon floats in the air.”
Then you’ll use an infrared sensor to detect the invisible fire.
Good idea, but the invisible fire is also heatless.”
You’ll spray-paint the dragon and make her visible.
“Good idea, but she’s an incorporeal dragon and the paint won’t stick.”
And so on. I counter every physical test you propose with a special explanation of why it won’t work.
Now, what’s the difference between an invisible, incorporeal, floating dragon who spits heatless fire and no dragon at all? If there’s no way to disprove my contention, no conceivable experiment that would count against it, what does it mean to say that my dragon exists? Your inability to invalidate my hypothesis is not at all the same thing as proving it true. Claims that cannot be tested, assertions immune to disproof are veridically worthless, whatever value they may have in inspiring us or in exciting our sense of wonder. What I’m asking you to do comes down to believing, in the absence of evidence, on my say-so.
Fallibilism opposes the conception of infallibilism which holds that empirical science can provide absolute knowledge, i.e. that there can be absolutely certain synthetic propositions. Roughly speaking, infallibilism was accepted until about the early 20th century. Ever since the times of Aristotle and all the way into the early 20th century it was generally assumed that at least some parts of empirical science had been established once and for all. However, this has been shown to be wrong time and time again. August Comte in the 19th century formulated the idea of a hierarchy of science (Cole, 1983). His idea was that science develops over time from the most general scientific discipline (sciences that deal with observable nature such as astronomy) to more complex systems. People started speculating about the stars and heavenly objects from the earliest of times and developed methods to predict eclipses and the weather. These developed into specifics about the nature of the physical worlds (physics) and the substances that make up the world (chemistry). Biology in this view is more complicated chemistry and psychology is even more complicated biology. The idea is that disciplines that are higher on this hierarchy are dependent on developments in their predecessors. We can see some evidence for that in how psychology developed in the late 19th century compared to physics which developed much earlier. More recent developments in psychology have involved brain imaging techniques that are based on developed within the fields of biology and physics.
This is one way of looking at the development of science. As we see in Figure 2.1, while astronomy is the basis of physics, it could also be placed at a higher level in the scientific hierarchy as describing objects in the universe that are bigger in scale than biological or geological systems. In either sense, we should not mistake the idea of complex science with “more difficult” or “better”. Complex in this context means how many phenomena need to be taken into account in understanding a system. Physicists can discuss subatomic particles and molecular bonds without reference to higher level entities such as animal cells. However, a biologist needs to consider chemical bonds and the physics behind them when studying biology while a psychologist needs to take into account the biology of the brain and nervous system.
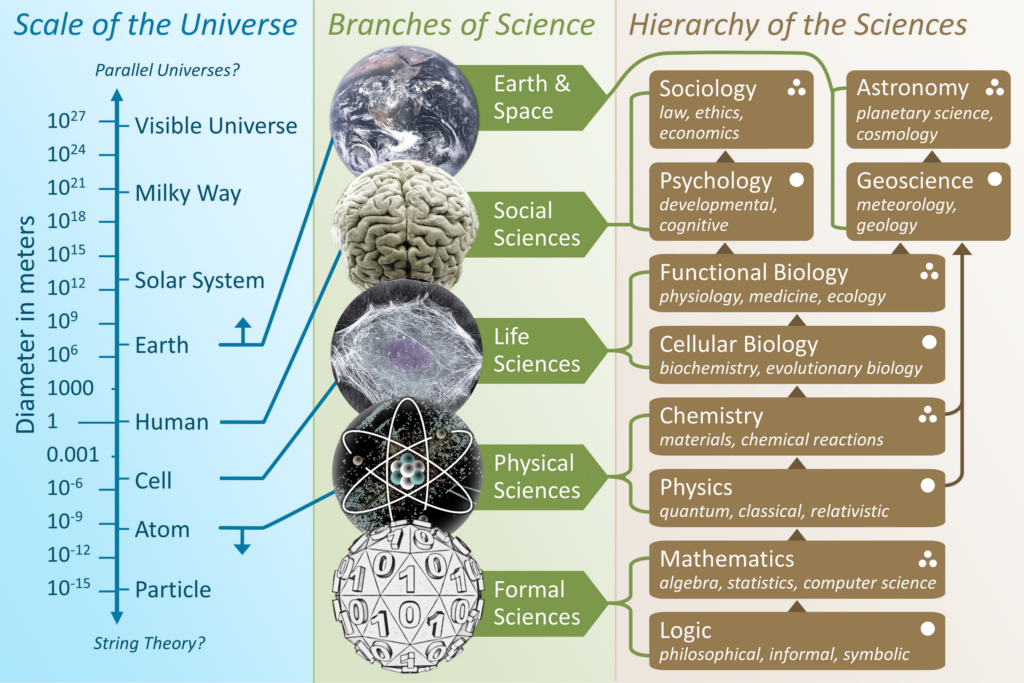
Philosophers would debate as to how exactly there can be absolutely certain synthetic propositions and which propositions of science have or haven’t been established beyond any reasonable doubt, but it was generally assumed that absolutely certain empirical knowledge is possible in one way or another. In the 18th and 19th centuries, the often-cited example of absolutely certain empirical theory was Newtonian physics. Around the time of its replacement with general relativity ca. 1920, it became clear that no empirical theory could ever be safe. That marked the transition to fallibilism. The following table will help summarize the debate between fallibilism and infallibilism:
Can there be absolutely certain synthetic propositions?
Yes | No |
Infallibilism: | Fallibilism: |
Synthetic propositions can be infallible. Empirical knowledge can be absolutely certain. | No synthetic proposition can be infallible. Empirical knowledge cannot be absolutely certain. |
👎 Problem of sensation | 👍 Problem of sensation |
👎 Problem of induction | 👍 Problem of induction |
👎 Problem of theory-ladenness | 👍 Problem of theory-ladenness |
The table begins with a formulation of the question at issue (the topic): can there be absolutely certain synthetic propositions? It then presents the two opposing answers to the question – the two conceptions (positions, viewpoints). Finally, the table lists the reasons (pros and cons) for and against these conceptions. The table shows that there are good reasons to accept the conception of fallibilism, which is exactly what contemporary philosophy does.
It is important to repeat that fallibilism doesn’t suggest that we have to stop relying on our senses, making inductive generalizations, or describing our observations by means of our accepted theories. In fact, all sciences still do this and that doesn’t impede their advancement. The point here is that because we make observations and generalizations, our synthetic propositions are inevitably fallible, i.e. they are not absolutely true.
This brings us to our answers to the two questions we formulated at the outset of the chapter.
- Can analytic propositions be absolutely certain? Yes.
- Can synthetic propositions be absolutely certain? No.
This outcome has serious consequences for both empirical and formal sciences. Since only analytic propositions can be absolutely true, absolute knowledge is only achievable in formal sciences, such as mathematics or logic. In contrast, absolute certainty is unachievable in empirical sciences, since empirical theories always contain synthetic propositions which, as we know, cannot be established beyond any reasonable doubt. Importantly, this is the reason why there cannot be such a thing as a proof in empirical science. Often scientists speak of having proven something in physics, or biology, or even social science, but strictly speaking proof is only achievable in formal sciences; empirical sciences don’t prove anything:
Formal Science | Empirical Science |
E.g. Logic, Mathematics, Systems Theory, game Theory, information Theory | E.g. Physics, Chemistry, Biology, Psychology, Sociology, Economics, Political Science |
Contains only analytic propositions | Contains synthetic propositions |
Absolute certainty is achievable | Absolute certainty is unachievable |
Propositions can be demonstratively proven | Propositions can only be confirmed by experience |
Now, if there is no such thing as a strict proof in empirical sciences, does this mean that every empirical theory is as good as any other? Are all competing empirical theories equally good, or is there a way to compare competing theories and find out which one of them is better? It is true, that all empirical theories are affected by the problems of sensations, induction, and theory-ladenness; all empirical theories without exception are fallible. Yet, we still find some of these empirical theories acceptable. Such ideas are fundamental to how scientists view the world. However, how do we educate ourselves and the public at large about which ideas or theories to accept? Do we all need to become philosophers of science to be able to engage with the world of science? Obviously, this is not the case. In the next chapter, we will discuss science and non-science in greater detail. Before we get there, let’s finish this chapter with a look at a toolkit that Sagan (1995) created to check whether something is empirically supported or not.
Carl Sagan’s Baloney Detection Kit
Think of any idea in modern discourse that is being discussed. Use the following suggestions in thinking about that idea.
- Wherever possible there must be independent confirmation of the “facts.”
- Encourage substantive debate on the evidence by knowledgeable proponents of all points of view.
- Arguments from authority carry little weight.
- Develop more than one hypothesis.
- Try not to get overly attached to a hypothesis just because it’s yours.
- If there’s a chain of argument, every link in the chain must work (including the premise) — not just most of them.
- Choose the hypothesis which requires the least number of assumptions to work.
- Always ask whether the hypothesis can be, at least in principle, falsified.